疼痛
Abstract
Past evidence has shown that motor cortical stimulation with invasive and non-invasive brain stimulation is effective to relieve cen-tral pain. Here we aimed to study the effects of another, very safe technique of non-invasive brain stimulation – transcranial direct cur-rentstimulation(tDCS–on pain control in patients with central paindue totraumatic spinal cord injury.Patients were randomized to receive sham or activemotor tDCS(2 mA,20 minfor 5 consecutive days).A blinded evaluator rated the pain using the visual analogue scale for pain,Clinician Global Impression and Patient Globa lAssessment.Safety was assessed with a neuropsychological battery and confounders with the evaluation of depression and anxiety changes.There was a significant pain improvement after active anodal stim-ulation of the motor cortex, but not after sham stimulation. These results were not confounded by depression or anxiety changes. Fur-thermore, cognitive performance was not significantly changed throughout the trial in both treatment groups. The results of ourstudy suggest that this new approach of cortical stimulation can be effective to control pain in patients with spinal cord lesion. We discusspotential mechanisms for pain amelioration after tDCS, such as a secondary modulation of thalamic nuclei activity.
? 2006 International Association for the Study of Pain. Published by Elsevier B.V. All rights reserved.
1. Introduction
One of the most refractory types of pain is the one associated with lesions of the brain or spinal cord, such as after stroke or traumatic spinal cord injury (Nichol-son, 2004). It is not uncommon that patients, despite the availability of multiple pharmacologic approaches,fail to experience sufficient relief, thus establishing the need for new therapeutic approaches and prompting a renewed interest in neuromodulatory approaches with brain stimulation. Deep brain stimulation has shown promising results (Wallace et al., 2004), but less invasive forms of stimulation might also be effective. Studies of epidural motor cortex stimulation report response rates that vary between 50% (Carroll et al., 2000) and 70–80%(Tsubokawa et al., 1993; Herregodts et al., 1995; Ebel et al., 1996; Nguyen et al., 1999; Nuti et al., 2005) and mean pain relief that varies from 28% to 47% in the larg-est series (Nguyen et al., 1999; Nuti et al., 2005) and 50% to 70% in the smallest series (Herregodts et al., 1995;Ebel et al., 1996). Non-invasive brain stimulation using repetitive transcranial magnetic stimulation (rTMS) also appears to be effective with mean pain relief in the range of 20–45% (Lefaucheur et al., 2004; Pleger et al., 2004;Khedr et al., 2005).
Another technique of non-invasive brain stimulation– transcranial direct current stimulation (tDCS) – might have further advantages over rTMS as it may lead to longer-lasting modulatory effects of cortical function,and is easy to administer and perform, less expensive,and provides a reliable sham-stimulation condition to assess the specificity of the effects.In tDCS, the cerebral cortex is stimulated through a weak DC current in a non-invasive and painless manner.Several studies have shown that this technique modu-lates cortical excitability in the human motor (Nitsche and Paulus, 2001) and visual cortex (Antal et al.,2001), that its modulatory effect remains after stimula-tion if tDCS is applied for several minutes, and that tDCS not only shifts activity of cortical areas situated directly under the electrodes, but also of distant areas,probably by interconnections of the primary stimulated area with these structures (Lang et al., 2005). Further-more, recent research has demonstrated that tDCS can enhance some aspects of cognition (Nitsche et al.,2003b; Fregni et al., 2005b; Iyer et al., 2005) and pro-mote the recovery of motor deficits in stroke patients(Fregni et al., 2005a; Hummel et al., 2005).
Therefore, here we aimed to study the effects of tDCS on pain reduction in patients with refractory central pain associated with spinal cord injury. We decided to study patients with spinal cord injury as pain develops in approximately 60–70% of these individuals, being severe in one-third of them (Bonica, 1991). In addition,because pain in these patients is associated with dysfunc-tional brain activity – a hyperexcitability of central noci-ceptors – rather than an overall increased input from peripheral pain receptors – a treatment that targets cen-tral structures might be the most adequate one in these patients.
2. Methods
2.1. Study design
Our study was a randomized, double-blinded, placebo-con-trolled, single-center, phase II parallel-group trial that was designed to evaluate the efficacy and safety of five daily ses-sions of transcranial direct current stimulation (tDCS) in patients with chronic neuropathic central pain due to traumat-ic spinal cord injury. Evaluation of safety was an important aim of the trial as previous safety studies of tDCS evaluated the effects of only one session of 2 mA in healthy volunteers or several sessions of 1 mA tDCS in patients with major depression (Fregni et al., 2005a; Iyer et al., 2005). The study conformed to the ethical standards of the Helsinki declaration(1964) and was approved by the institutional ethics committee.All patients provided written, informed consent.
2.2. Subjects
Patients were prospectively and sequentially selected from a specialized pain clinic in the rehabilitation medical center of the Sao Paulo University. They were regarded as suitable to participate if they fulfilled the following criteria: (1) traumatic spinal cord injury – for instance, due to fall, car accident or gun shot; (2) stable chronic pain for at least the three preceding months; (3) score higher than or equal to 4 cm (0 cm = ‘no pain’ and 10 cm = ‘worst possible pain’) on the visual ana-logue scale (VAS) for pain perception at the baseline/start of the treatment; (4) refractoriness to drugs for pain relief – such as tricyclic antidepressants, antiepileptic drugs, and/or narcot-ics (pain resistant to at least two of these drugs supplied in ade-quate dosages for 6 months) and (5) pain was not attributable to other causes, such as peripheral inflammation.
We excluded patients with any clinically significant or unstable medical or psychiatric disorder, history of substance abuse, or neuropsychiatric comorbidity. In addition, we excluded patients with implanted devices for pain control, such as vagal or deep brain stimulators.Patients with clinical signs of syringomyelia were investigated with MRI and excluded if the MRI was positive for this condition. Only patients without significant alterations in head computed tomography and/or head MRI were included in the study. Seventeen patients(mean age 35.7 ± 13.3 years, mean ± SD, 3 females) partici-pated in the study. The general clinical characteristics of the patients are summarized in Table 1. Note that the low preva-lence of females in the study is due to the low prevalence of females with spinal cord injury in our population (Sao Paulo,Brazil). Because a large number of spinal cord injuries occur in construction workers (work related – fall) or as a result of gun shots, prevalence of traumatic spinal cord injury is higher in men (Barros Filho et al., 1990).
2.3. Experimental design
The study had two phases: (1) baseline evaluation – that consisted in a 2-week period of observation to establish a base-line for pain and the other measures (such as mood, anxiety,and cognition) – this period of evaluation was necessary for habituation with daily measurements and to capture variability in the baseline pain scores; (2) 3-week double-blinded treat-ment – that consisted in daily treatment sessions with sham or active tDCS for 5 consecutive days and a follow-up period of 16 days.
During the baseline period, patients were randomized in a 1:2 ratio to receive sham or active tDCS, respectively. This randomization strategy (1:2) was chosen, given the relatively small sample size planned for this phase II trial and the lack of a priori information on the efficacy for a new treatment
(see (Peto, 1978)). Randomization was performed using the order of entrance in the study and a previous randomization list generated by a computer using random blocks of six (for each six patients, two were randomized to sham and four to active tDCS) in order to minimize the risk of unbalanced group sizes.
To determine the sample size, we used the results of a pre-vious study (Lefaucheur et al., 2004) that investigated rTMS for the treatment of central pain, because no studies are avail-able for the treatment of pain with tDCS. In this study, a mean reduction of pain of 22.9% (±3.6) in the active group and 7.8%(±3.2) in the sham group was reported. Considering a power of 97.5% (this represents a power of 95% considering an unequal randomization – see (Pocock, 1979)) and a critical alpha = 0.01%, eight patients (four in each group) are needed to detect group differences. As we had no direct knowledge about the effect size of tDCS, we decided to increase the num-ber of subjects studied to 14. To account for possible dropouts,we added three more subjects. Therefore, 17 patients were entered into the study.
2.4. Direct current stimulation
Direct current was transferred by a saline-soaked pair of surface sponge electrodes (35 cm 2 ) and delivered by a specially developed, battery-driven, constant current stimulator(Schneider Electronic, Gleichen, Germany) with a maximum output of 10 mA. Patients received either anodal stimulation of primary motor cortex (M1) or sham stimulation of M1.For anodal stimulation of M1, the anode electrode was placed over C3 or C4 (EEG 10/20 system) and the cathode electrode over the contralateral supraorbital area. This electrode posi-tion has been shown to be effective to enhance excitability of the primary motor cortex (Nitsche and Paulus, 2001). The hemisphere of stimulation was based on pain lateralization.For patients with an asymmetric pain, we stimulated the con-tralateral M1 – similar to the methodology of Lefaucheur's study (Lefaucheur et al., 2004) and for patients with symmetric pain, we stimulated the dominant, left (for right-handed patients) hemisphere. However, it should be noted that M1 stimulation with tDCS induces widespread changes in the activity of cortical areas and can indeed change the activity of the contralateral motor cortex (see Lang et al., 2005). This suggests that unilateral tDCS treatment might be sufficient for patients with bilateral pain. In addition, because the elec-trode for tDCS is large and the longer axis was aligned with the motor cortex strip, the stimulation encompassed a broad area of the motor cortex (upper limb and part of the lower limb and face) – see Fig. 1 in which we measured the brain location of the electrode using a frameless stereotactic system(Brainsight, Rogue Research, Montreal, Canada). A constant
current of 2 mA intensity (subthreshold intensity) was applied for 20 min. Stimulation with 2 mA (for a single session) has been shown to be safe in healthy volunteers (Iyer et al.,2005).Subjects felt the current as an itching sensation at both electrodes at the beginning of the stimulation. For sham stim-ulation, the electrodes were placed in the same positions as for anodal M1 stimulation; however, the stimulator was turned off Fig.
1.Illustration showing the electrode area over the motor cortex.The position of the electrode was determined using a frameless stereotactic system (Brainsight software, Rogue Research, Canada).Note that the electrode covers almost the entire motor cortex strip.
after 10 s of stimulation as previously described (Fregni et al.,2005a) and also assessed as a reliable method of sham stimula-tion (Fregni et al., in press-b). Therefore, the subjects felt the initial itching sensation, but received no current for the rest of the stimulation period.
2.5. Evaluations and endpoints
All evaluations were performed by a blinded rater twice at baseline, daily during treatment period (except BDI, MMSE,and cognitive tests, that were conducted on day 1 and day 5,and CGI and PGA that were measured on day 2, 3, 4, and 5), and once in the follow-up visit. Except for VAS pain and VAS anxiety (that were carried out before and after stimula-tion), all evaluations were carried out immediately after the stimulation.
2.5.1. Pain measurement
Visual analogue scale (VAS) for pain. This self-evaluation scale ranges from 0 to 10 as visually described in centimeter units: 0 cm indicates no pain and 10 cm means the worst pain possible. Participants were asked to rate their pain dur-ing the previous 24 h. This scale has been widely used in studies that evaluate pain as an outcome: both validity and reproducibility have been demonstrated (Bolton and Wilkin-son, 1998). As we expected daily variability in pain levels,pain was self-assessed frequently during baseline (once a day for 2 weeks) and then twice a day during the treatment phase (before and after the treatment) and once at the fol-low-up period.
Analgesic use. We instructed the participants to continue their routine medication regimen. All changes in dosing were recorded in the patient’s medication use diary.
Herbal remedies and alternative therapies such as massage or acupuncture were allowed if they had been used for at least 4 weeks before randomization, and the regimen was main-tained constant throughout the study.
Clinical Global Assessment (CGI). CGI is a two-item scale that encompasses: (1) Severity of Illness and (2) Global Improvement.Item 1 is rated on aseven-pointscale(1 = normal to 7 = extremely ill); item 2 on a seven-point scale (1 = very much improved to 7 = very much worse). The severity of illness item requires the clinician to rate the severity of the participants’ illness at the time of assessment as relative to the clinician’s past experience with participants who have the same diagnosis. The Global Improvement item requires the clinician to rate how much the participants’illness has improved or worsened relative to a baseline state.
Patient Global Assessment (PGA). The Global Assessment asks each participant to rate the change in his/her overall pain status relative to their personal baseline on a seven-point scale ranging from much worse to much improved. Although the PGA provides a retrospective recording that may overestimate improvement as a result of recall or motivational bias, we con-sider this scale to be important as it is easy for the participants to understand and yields data that correspond to the main treatment target (pain symptom severity).
2.5.2. Confounders measurement
Because depression and anxiety can be important con-founders for pain improvement, we measured these two domains using the following instruments:
Beck Depression Inventory (BDI). The Beck Depression Inventory (BDI) is a 21-item test presented in multiple-choice format, which measures the presence and the degree of depres-
sion in adults.
VAS for anxiety. The VAS for anxiety is a self-evaluation scale that ranges from 0 to 10 cm, where 0 means no anxiety and 10 cm means the worst anxiety ever.
2.5.3. Cognitive function and safety
Although the electric current induced by tDCS is weak(2 mA – well below the pain threshold), it is applied continu-ously for several minutes in this protocol. Since potential adverse effects of this technique might include cognitive chang-es, we completed the following tests:
Mini-mental state examination. This is a brief, quantitative measure of cognitive status that is widely used to examine cogni-tion at a given point of time and to follow the course of cognitive changes in an individual over time (van Belle et al., 1990).
Stroop test. This instrument is a test of selective attention and interference susceptibility. This test might measure cogni-tive domains that involve focused attention and decision-mak-ing, and thus provide a measure of executive (frontal) function.Digit span forward and backward. These two tests are rela-tively simple to apply and provide valuable information about attention, information storage, working memory, and mental double-tracking and reversing operations.
Simple reaction time. Because our stimulation was targeted to motor cortex, assessing a possible detrimental effect of anodal tDCS on motor function was important. We used a
simple reaction time task in which patients were seated in front of a computer screen placed at eye level. A circle of 4 cm diameter was presented in the center of the screen following a warning sign (small cross in the center of the screen) after a randomly variable interval of 2–5 s. The patients were asked to push a response key as soon as they saw the circle on the screen using only the right index finger (afterwards the test was repeated with the left index finger), which was rested on the key. The time between the appearance of the circle and the push of the response key was defined as simple reaction time(sRT). The experiment consisted of blocks of 30 trials. Patients were allowed to practice for 60 trials prior to the test in order to familiarize themselves with the procedure. The stimuli were generated and response times recorded using Superlab pro v2.0 software (Cedrus Corporation, San Pedro, CA).Finally we monitored adverse events, asking patients after each session of stimulation and in the follow-up if they have experienced any adverse event and the relationship of these events to the treatment with tDCS.
2.5.4. Statistical analysis
Initially we compared baseline results for both groups of patients in order to rule out that differences between sham and active stimulation might have been due to a priori differ-ences between these two groups. For this analysis, continuous variables were compared using Student’s t-test and categorical variables using Fisher’s exact test. Analyses were done with Stata statistical software (version 8.0, College Station, Texas).Dropouts in our study might indicate a treatment failure as these patients depend on special transportation to come to the hospital to receive the treatment and have a low socio-economic status so that no improvement would discourage their contin-uation in this trial. Therefore, we analyzed the primary and secondary endpoints using the intention-to-treat method including patients who received at least one dose of the ran-domized treatment and had at least one post-baseline efficacy evaluation. We used the last evaluation carried out to the ses-sion before the missed session, assuming no further improve-ment after the dropout, for this calculation.
For the analyses with continuous variables, we used a repeated-measures analysis of variance in which the dependent variable was the endpoint (for instance VAS scores) and the factors were: group (sham and active tDCS) and time of treat-ment (baseline, day 1, day 2, day 3, day 4, day 5, and follow-up or baseline, day 5 and follow-up). In addition, for some end-points, we also ran a one-way ANOVA of the active tDCS group including only the time factor as a repeated measure-ment. When appropriate, post hoc comparisons (between sham and active group and between post-treatment evalua-tions and baseline evaluations) were carried out using Bonfer-roni correction for multiple comparisons.
For categorical variables, we constructed a 2 · 2 table with group of treatment (sham or active) as a row and time of treat-ment (before and after 5 days) as column and performed a measure of association using Fisher’s exact test.
To evaluate if depression and anxiety were confounding our results, we built a model of regression. Pain improvement was taken as dependent variable. We included the independent variables time, group, and time · group. The variables depres-sion (as defined by BDI score changes) and anxiety (as defined by VAS anxiety score changes) were added individually to the
model. Confounding was defined by changes of ±20% or higher in the beta coefficient of the interaction term of the original(reduced) model.
Finally, we correlated pain change (as indexed by change in VAS score) with some disease characteristics, such as disease duration, baseline VAS pain scores, site of spinal cord lesion(lumbar, thoracic or cervical), Frankel classification (complete versus incomplete lesion), type of pain (paroxysmal, continu-ous or both), and location of pain (lower limbs, upper limbs
or both). Pearson correlation coefficient was used to analyze the relationships between continuous variables. For categori-cal variables we dichotomized the mean pain changes in two groups and compared them using a Student’s t-test.
Unless stated otherwise, all results are presented as means and standard deviation, and statistical significance refers to a two-tailed p value < 0.05.
3. Results
Table 1 summarizes demographic and clinical char-acteristics at baseline and Table 2, medication character-istics at baseline. There were no significant differences in clinical and demographic characteristics between the sham and active tDCS groups. All patients tolerated the tDCS well without experiencing any adverse effects.When explicitly asked, none of the patients could tell whether the stimulation was active or sham. There were two dropouts – one in each group – and the main reason that prevented both patients to complete the experiment was the difficulty in transportation. Additionally, both patients had no or small improvement that could have contributed to their decision of abandoning the treatment.
Patients described their pain as: a burning (76.5%),stabbing (58.9%) or tingling sensation (35.3%). The most common site of pain were the lower limbs (64.7%), followed by back pain (29.4%), and pain in the upper limbs (17.6%). Only one patient reported also pain in the face (5.8%). Pain was described as continu-ous (41.2%) or continuous with paroxysms (41.2%), or only paroxysmal (17.6%).
Routine medical treatment of the patients was per-formed according to the guidelines of our pain clinic.They therefore were using the following medications:amitryptiline (low dosage – 25 to 75 mg/day – 58.8% of patients); antiepileptic drugs (carbamazepine or gaba-pentin – 41.2%); baclofen (41.2%); neuroleptic (chlor-promazine – 23.5%); benzodiazepines (diazepam and clonazepam – 29.4%); one patient only was taking mor-phine (5.8%).
3.1. Pain control – VAS
To analyze whether tDCS treatment was associated with pain improvement, we performed a repeated-mea-sures analysis of variance in which the dependent vari-able was change of VAS pain scores from the baseline and the independent variable was time of evaluation(baseline, day 1, day 2, day 3, day 4, day 5, and fol-low-up) and group of treatment (active versus sham tDCS). This analysis revealed a significant main effect of group of treatment (F (1,105) = 61.1; p < 0.0001) and interaction term (group versus time; F (1,105) = 3.03;p = 0.009) (Fig. 2A). We therefore performed addition-ally a one-way ANOVA to examine the main effect of time in the active group only. Because this effect was sig-nificant (F (6,30) = 11.95; p < 0.0001), we performed post hoc comparisons using Bonferroni correction (compar-ing baseline VAS scores with the subsequent sessions).This analysis showed a significant difference after the third session that remained significant until the last ses-sion for the active tDCS group (Baseline comparison with: first session – p = 0.95; second session – p = 0.1;third session – p = 0.015; fourth session – p = 0.001;fifth session – p = 0.001, corrected for multiple compar-isons). In the follow-up, however, there was only a trend toward a significant difference between baseline versus follow-up (p = 0.1). The largest pain reduction was achieved after the fifth session of stimulation (mean
VAS pain scores – 2.6; 95% confidence interval (CI),1.44–3.74). The magnitude of this effect decreased dur-ing the follow-up period (mean VAS pain scores – 3.9;95% CI, 2.6, 5.1), however, pain rating was still dimin-ished compared to baseline scores (mean VAS pain scores – 6.2; 95% confidence interval (CI), 5.1–7.3).Because for the sham tDCS group, the one-way ANOVA showed no time effect (F (6,30) = 1.12;p = 0.37), we did not perform post hoc comparisons for the different time points. And it is worthy of note that the magnitude of the placebo effect was indeed low compared to other studies (varied from ?18.9% to
+9.8% during treatment). Such low placebo response may indicate the clinical refractoriness of our popula-tion, since it has been shown that the severity and ther-apeutic refractoriness of symptoms do correlate negatively with placebo response (Ruck and Sylven,2006).
Interestingly, not only the effects of consecutive ses-sions were cumulative, but they also lasted for at least 24 h as the pain VAS scores for the post-treatment were not significantly different from those of the pre-treat-ment on the following day (see Fig. 2B) (p = 0.93,p = 0.80, p = 0.76, p = 0.25, for the comparisons T1 after/T2 before; T2 after/T3 before; T3 after/T4 before and T4 after/T5 before, respectively) (Fig. 2B).
We compared VAS changes at each time point between sham tDCS and active tDCS. This analysis showed similar results as the comparison of the active tDCS group relative to baseline. The difference between active and sham tDCS treatment became significant after the second session (first session – p = 0.18; second session – p = 0.047; third session – p = 0.039; fourth ses-sion – p = 0.028; fifth session – p = 0.004, corrected for multiple comparisons) and the effects decreased in the last session as there was only a trend for a difference between the two groups at this time (p = 0.063).
There were seven responders (reduction of 50% or more in the VAS) in the active tDCS group (63% of patients) and only 1 responder in the sham tDCS group(16%). This difference was marginally significant(v 2 = 3.68; p = 0.055). Interestingly, in the follow-up evaluation; four patients in the active tDCS group were still considered as responders and the only responder in the sham tDCS group reported no longer pain improve-ment, there was a trend for a significant difference between these two groups at this time point (v 2 = 2.85;p = 0.09).
3.2. Pain control – CGI and PGA
We initially performed repeated-measures ANOVA in which we included two factors: group of treatment(active or sham tDCS) and time of evaluation (day 2,day 3,day 4,day 5,and follow-up). This analysis revealed an effect of group (F (1,75) = 54.1; p < 0.0001),but not time (F (4,75) = 0.65; p = 0.65), or interaction time · group (F (4,75) = 1.11; p = 0.35), suggesting that
the treatment-related difference in both groups was sta-ble throughout the study (i.e., independent of time).Because these tests do not contemplate baseline values as they measure clinical improvement over time, this might have decreased the power to detect a significant
interaction. Thus, we performed an individual repeat-ed-measures ANOVA for the active group only and found a trend toward a significant effect of time (F (4,40) = 2.38; p = 0.068).
We performed a similar analysis for PGA and obtained similar results. The overall ANOVA for this analysis revealed an effect for group (F (1,75) = 42.28;p < 0.0001), but not for time (F (4,75) = 0.88; p = 0.48),or interaction time · group (F (4,75) = 0.80; p = 0.53).An individual repeated-measures ANOVA for the active group only revealed a significant effect of time(F (4,40) = 3.31; p = 0.02), suggesting that there was a sig-nificant trend of greater pain reduction (as indexed by PGA) over time in the active tDCS group.Finally, we performed a post hoc analysis comparing the sham and active tDCS groups at the different time-points. For the CGI, there was a significant difference between active and sham tDCS groups on day 2(p = 0.042), day 3 (p = 0.017), day 4 (p = 0.013), day 5(p = 0.012), but not in the follow-up (p = 0.22). For the PGA, a significant difference was observed only on day 3 (p = 0.033) and day 4 (p = 0.013); there was a trend for a significant difference on day 5 (p = 0.07) and follow-up (p = 0.1); but not for day 2 (p = 0.57)(see Figs. 3A and B). Note that these tests were not per-formed for day 1 as they measure the clinical improve-ment over a period of time.
3.3. Anxiety and depression
For anxiety, we initially performed a repeated-mea-sures ANOVA with two factors: time (day 1, day 2,day 3,day 4,day 5 and follow-up) and group (active and sham tDCS). This analysis revealed a significant effect of time (F (6,105) = 3.95; p = 0.001), but not of group (F (1,105) = 0.63; p = 0.42) or the interaction time · group (F (6,105) = 0.14; p = 0.99). This result sug-gests that there was a change of anxiety levels through-out the trial that was similar in both groups (see Fig. 4).
We performed a similar analysis for depression eval-uation – BDI scale scores. This analysis showed no sig-nificant effect of time (F (2,45) = 0.20; p = 0.82), group of treatment (F (1,45) = 0.63; p = 0.43) or interaction term time · group (F (2,45) = 0.06; p = 0.94). The mean BDI scores at baseline were 9.3 (±6.7) in the active tDCS group and 11.3 (±5.4) in the sham tDCS group and after 5 sessions of treatment they were 8.8 (±5.4) and 9.5 (±4.1), respectively.
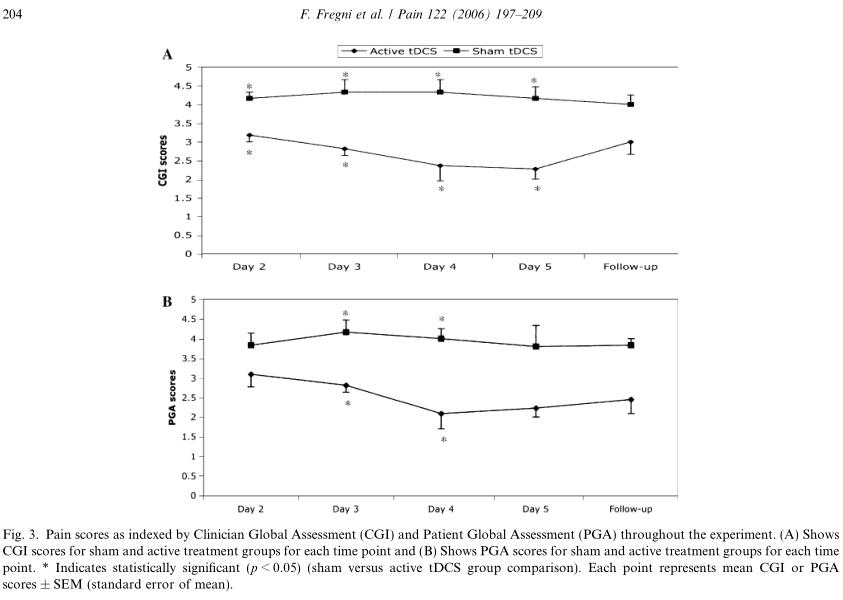
Although there was no significant effect of the treat-ment on depression and the effects of tDCS on anxiety were similar in both groups, we calculated two regres-sion models in which pain improvement was the depen-dent variable in order to further rule out changes in anxiety and mood as confounders for the measured pain improvement. In the first model, we included the follow-ing variables: time, group, time · group, and anxiety (or depression). In the second model (reduced model), we included only time, group, and time · group. For anxi-ety this analysis showed that the beta coefficient for the interaction term in the reduced model was 0.69(p < 0.001) and the inclusion of the term anxiety did not change it by more than 20% (0.65, p < 0.001), showing thatanxietywasnotconfoundingourresults.For depres-sion,the same analysis showed that the beta coefficient for the interaction term in the reduced model was 0.65(p = 0.01) and the inclusion of the term depression did not changeit by more than 20%(0.66,p = 0.008),showing that depression was not confounding our results.
Finally it should be noted that the lack of effects of tDCS on depression and anxiety symptoms might be due to the stimulated area (motor cortex) and mild symptoms of both depression and anxiety in this patient group.
3.4. Safety – cognitive and motor evaluation
The analysis for the MMSE showed that there was no significant effect for the main effect of time (F (2,45) =0.27; p = 0.76), group of treatment (F (1,45) = 0.59;p = 0.44) or interaction term time · group (F (2,45) =0.92; p = 0.40), therefore MMSE scores did not change significantly throughout the trial. The mean MMSE scores at baseline were 24.5 (±2.2) in the active tDCS group and 25.5 (±3.7) in the sham tDCS group and after five sessions of treatment they were 24.8 (±2.7) and 26.3(±3.6), respectively.
For the Stroop test, we collapsed and averaged the results of performance of the Stroop colors, words,and interference task, as these were similar. A repeat-ed-measures ANOVA with two factors: group (active and sham tDCS) and time (baseline, day 5 and fol-low-up) showed no significant main effect of group(F (1,45) = 2.34; p = 0.13), time (F (2,45) = 0.25; p = 0.78),and the interaction group · time (F (2,45) = 0.001;p = 0.99).Interestingly, although there was no signifi-cant time effect, both groups showed a negative slope(Fig. 5A) (i.e., a decrease of the execution time over these three time points) indicating a possible learning effect.
For the analysis of the digit span forward and back-ward (DSF and DSB) performance, we performed a similar analysis. The effects for group of treatment(F (1,45) = 0.01; p = 0.90 for DSF and F (1,45) = 1.60;p = 0.21 for DSB), time (F (1,45) = 0.29; p = 0.75 for DSF and F (1,45) = 0.26; p = 0.77 for DSB), and the inter-action time · treatment (F (1,45) = 0.001; p = 0.99 for DSF and F (1,45) = 0.35; p = 0.71 for DSB) (see Figs.5B and C) were not significant.
Finally, for the simple reaction time test, we initially performed a repeated-measures ANOVA that showed no significant effect for group of treatment (F (1,30) =1.60; p = 0.22), time (F (2,30) = 0.37; p = 0.69), and the interaction time · treatment (F (2,30) = 0.27; p = 0.76)for the right hand, and similar results for the left hand(F < 1 for the group, time, and interaction effect) (see Table 3 for the summary results at each time point).Although we did not show a significant difference in motor performance after treatment between the two treatment groups, a respective trend can be seen:whereas there was a motor improvement of 23.6% in the active group, patients in the sham group had a wors-ening of 2.3% of motor function. The lack of signifi-cance may be a result of the small sample size(aggravated by the fact that some patients could not per-form this task) and the variance of the baseline motor function across patients.
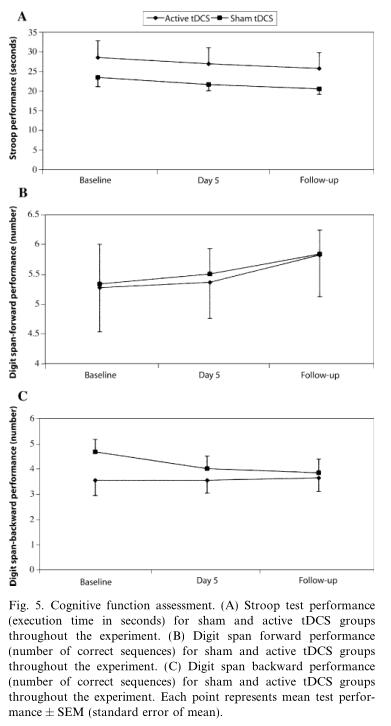
Fig. 5. Cognitive function assessment. (A) Stroop test performance(execution time in seconds) for sham and active tDCS groups throughout the experiment. (B) Digit span forward performance (number of correct sequences) for sham and active tDCS groups throughout the experiment. (C) Digit span backward performance(number of correct sequences) for sham and active tDCS groups throughout the experiment. Each point represents mean test perfor-mance ± SEM (standard error of mean).
3.5. Adverse effects
There was a low incidence of adverse events in both groups of treatment. These adverse events were all mild and consisted of mild headache (three in the active group and one in the sham group) and itching under the electrodes (two in the active group and three in the sham group).Importantly, active tDCS did not worsen pain in any of the patients that received this treatment.
3.6. Correlations – pain improvement and disease characteristics
Although the small sample size of this study limits the investigation of potential predictors associated with a good outcome (for the active tDCS group only), we performed correlation analysis for some characteristics of the disease. This analysis revealed a significant nega-tive correlation between pain improvement and duration of disease (r = ?0.66, p = 0.025) – which suggests that the shorter the disease duration, the larger is the pain improvement. Pain improvement and baseline VAS scores did not correlate significantly (r = ?0.11,p = 0.74) (Fig. 6). To compare the categorical variables with pain improvement, we dichotomized patients in two groups and compared whether the mean pain reduc-tion was different between both groups. This analysis revealed that patients with pain paroxysms (compared to continuous pain only) had a significantly greater reduction in their pain scores (D = 4.74 ± 0.95 versus D = 1.12 ± 1.54, respectively, p = 0.005). In addition,pain in the lower limbs and thoracic and lumbar lesions were significantly associated with better outcome com-pared to pain in the upper limbs/back pain and cervical lesions (D = 4.79 ± 0.83 versus D = 1.1 ± 1.3, respec-tively, p = 0.0006, for the comparison of pain location and D = 4.99 ± 1.00 versus D = 1.16 ± 1.09, respective-ly, p = 0.024 for the comparison of lesion site). More-over, there was no significant difference in pain improvement between the groups of patients with com-plete lesion versus patients with incomplete lesion (Fran-kel classification, p = 0.27).

Because carbamazepine might decrease the effects of anodal stimulation after a single session of tDCS (Lie-betanz et al., 2002), we did a subanalysis in which we divided patients by use of antiepileptic drugs (carbamaz-epine (two patients); gabapentine (two patients) and no antiepileptic drugs (seven patients)). Although there was a difference in the magnitude of the effects across the three groups (mean pain reduction of 36.7%, 62.9%,and 74.1%, respectively), they were not significantly dif-ferent (perhaps due to the small sample size of the sub-groups). However, this result might suggest that patients with carbamazepine may respond less to this treatment.
4. Discussion
4.1. Pain improvement
Previous studies showed that high-frequency rTMS –another technique of non-invasive brain stimulation – is also associated with a significant pain improvement compared to sham stimulation (Lefaucheur et al.,2001, 2004; Pleger et al., 2004; Khedr et al., 2005). Inter-estingly the attempt of the use of inhibitory low-frequen-cy motor cortex rTMS (1 Hz) for pain control in these patients did not result in pain alleviation (Lefaucheur et al., 2004). Based on these studies, it has been pro-posed that an increase of the local excitability of the motor cortex might be associated with pain control in patients with central pain. The mechanism of tDCS to reduce pain in our patients might be similar, because anodal tDCS is also associated with an increase of cor-tical excitability that lasts beyond the stimulation period(Nitsche and Paulus, 2001; Nitsche et al., 2003a). How-ever, the mechanisms responsible to increase cortical excitability might be different between tDCS and rTMS as tDCS induces a weak continuous electric current and rTMS (and also epidural stimulation) induces repetitive pulses of electrical current at near threshold intensities.It has been proposed that tDCS induces a change in the electrical properties of the neuronal membrane that can result in a hyperpolarization or depolarization of the stimulated area (Nitsche et al., 2005); while repetitive stimulation induced by rTMS induces a change in the synaptic strengthening efficacy through mechanisms of long-term potentiation and depression (Huang et al.,2005). The main difference with regard to the after-ef-fects of both techniques might be that tDCS induces a more widespread and long-lasting effect compared to rTMS (Nitsche and Paulus, 2001; Romero et al., 2002;Lang et al., 2005). However, both techniques might lead to a similar indirect change of activity of connected areas and thus result in similar effects on pain.
Up-regulation of motor cortex excitability might modulate pain perception through indirect effects on pain-modulating areas, such as thalamic nuclei. Indeed,a change of the thalamic and subthalamic nuclei activity is associated with epidural stimulation of the motor cor-tex (Peyron et al., 1995; Garcia-Larrea et al., 1997,1999), TMS (Strafella, 2004) and tDCS (Lang et al.,2005). Garcia-Larrea et al. (1997) proposed a model in which thalamic nuclei activation would lead to several events in other pain-related structures, such as the ante-rior cingulate, periaqueductal gray, and spinal cord that could ultimately modulate the affective-emotional com-ponent of pain and also inhibit pain impulses from spinal cord (Garcia-Larrea et al., 1997, 1999).
An important consideration is the functional reorga-nization of the motor cortex after spinal cord injury.Two studies showed a significant correlation between changes in the motor cortical representation and phan-tom limb pain (Flor et al., 1998; Karl et al., 2001). If such plastic changes following deafferentation are caus-ally involved with chronic pain, then somatotopic stim-ulation would be advantageous. However, the current clinical data are paradoxical. Whereas the best stimula-tion site using rTMS for pain control is not the area cor-responding to the painful zone (but the adjacent one)(Lefaucheur et al., 2004), for epidural stimulation, a study with 32 patients showed the most efficacious posi-tion of the stimulating electrodes was the one corre-sponding to the cortical somatotopic representation of pain perception (Nguyen et al., 1999). For tDCS, due to the large electrode size, it is likely that it induced a modulation over a large area of the motor cortex that encompassed both the area corresponding to the pain and the adjacent area (see Fig. 1). Further studies are needed to explore whether smaller electrodes with somatotopically guided stimulation might optimize the analgesic effect of tDCS.
Compared to previous studies that used brain stimu-lation for the treatment of chronic pain, the magnitude of our results (mean pain response of 58%) is superior to that of other similar rTMS studies that report mean pain relief that ranges from 20 to 45% (Lefaucheur et al., 2001; Khedr et al., 2005); however, when analyz-ing the response rate, it is a little inferior than Khedr’s study (63% versus 75%) – that had the largest response rate. Regarding the long-lasting effect, only one study investigated the effects of five consecutive sessions of rTMS – Khedr’s study. The comparison of long-lasting effects between Khedr’s and our study showed similar results: our study reports a mean pain reduction of 37% in the follow-up evaluation – that is similar to the 40% decrease in Khedr’s study. Although there is a dif-ference in the percentage of pain responders (36% versus 61%, ours and Khedr’s study, respectively), this might be a result of the different criteria of pain responders between these studies (i.e., whereas the threshold for responders was set at 50% in our study, this was 40% in Khedr’s study). Finally, compared to epidural stimu-lation, tDCS may result in a smaller treatment effect (our study showed 58% mean pain decrease and 63% of responders versus surgical approach that showed 28–70% of mean pain reduction and 50–80% of respond-ers). The main reason for this difference is that the effects of five sessions of tDCS are likely to have a shorter impact on brain activity than what is achieved using direct motor cortex stimulation with epidural electrodes for several months. Although there is a difference in the magnitude of the pain improvement across these differ-ent techniques of brain stimulation, previous data sug-gest that patients that respond to epidural stimulation also respond to noninvasive brain stimulation with rTMS (Migita et al., 1995; Canavero et al., 2002). Fur-ther studies should also test whether tDCS treatment can be a predictor to the effects of epidural stimulation for the treatment of pain.
One interesting finding of previous studies is the rela-tionship between sensory changes and therapeutic improvement after motor cortex stimulation (Drouot et al., 2002; Brown and Pilitsis, 2005), however, we did not find a significant relationship between pain improve-ment and sensory deficits (as defined by Frankel classifi-cation) – one of the reasons might be because of the small sample size of this study and low number of patients with some degree of intact sensory function(three in the active and three in the sham tDCS group).
4.2. Safety – cognitive and motor evaluation Because tDCS has been only recently tested for clin-ical applications, such as stroke (Fregni et al., 2005a;Hummel et al., 2005) and depression (Fregni et al., in press-a), the impact of consecutive sessions of motor cortex tDCS (at the intensity of 2 mA) on cognition and specifically on the motor function is not yet fully known.Our findings show no significant cognitive and motor performance change in either group after the treatment with sham or active tDCS.
These results are in line with the study by Iyer et al.(2005) on the safety of tDCS. In this study, the authors tested the safety of tDCS using a neurocognitive battery and motor tests (simple reaction test and Pegboard Grooved test). The authors did not find any significant cognitive or motor deterioration with tDCS application, on the contrary, they found a significant improvement of verbal fluency after stimulation with anodal tDCS over the prefrontal cortex. They are also in accordance with other safety studies using related stimulation proto-cols, where no change of serum neurone specific enolase concentration – a sensitive marker of neuronal damage,no disturbances of the blood–brain-barrier and no cere-bral edemas were found (Nitsche and Paulus, 2001; Nit-sche et al., 2003a, 2004). The results of our study,therefore, add more safety information as we showed that there was no cognitive or motor function worsening after five consecutive days of 2 mA motor cortex anodal stimulation. To our knowledge, this parameter of stim-ulation (2 mA for 5 consecutive days) is the highest dose of tDCS applied in clinical trials thus far (higher than our depression study that used 1 mA of tDCS over 5 consecutive days) (Fregni et al., in press-a). However,we did not measure other safety parameters such as brain activity using electroencephalogram and therefore our safety data should be interpreted as a preliminary safety evidence.
4.3. Limitations
This study has some limitations that should be dis-cussed. Although we selected patients with central pain due to traumatic spinal injuries only, the localization of the lesion and etiology of trauma differed across patients and our sample size might not have been large enough to detect characteristics associated with a posi-tive outcome. Second, a potential limitation was the fact
that patients were allowed to change their medication regimen throughout the trial; however, we did not detect a significant change in the use of these medications, per-haps due to the fact that most of the patients were tak-ing drugs with chronic effects such as antidepressants.Therefore, medication change did not confound our results.
Acknowledgments
This work was supported by a grant from the Har-vard Medical School Scholars in Clinical Science Program (NIH K30 HL04095) to F.F. A.P.-L. is supported by K24 RR018875, RO1-EY12091, RO1-DC05672, RO1-NS 47754, RO1-NS 20068, and R01-EB 005047. The authors are thankful to Barbara Bonnetti for the help on the coordination of this study and Marco Antonio Marcolin for the administrative support.
References
Antal A, Nitsche MA, Paulus W. External modulation of visual perception in humans. Neuroreport 2001;12:3553–5.
Barros Filho TE, Taricco MA, Oliveira RP, Greve JM, Santos LC,Napoli MM. Epidemiological study of patients with spinal cord injuries and neurologic deficit, admitted to the Institute of Orthopedics and Traumatology at the Hospital das Clinicas of the School of Medicine of the University of Sao Paulo. Rev Hosp Clin Fac Med Sao Paulo 1990;45:123–6.
Bolton JE, Wilkinson RC. Responsiveness of pain scales: a compar-ison of three pain intensity measures in chiropractic patients.J Manipulative Physiol Ther 1998;21:1–7.
Bonica JJ. Introduction: semantic, epidemiologic, and educational issuers. In: Casey KL, editor. Pain and central nervous system disease:thecentralpainsyndromes. NewYork:RavenPress;1991.
Brown JA, Pilitsis JG. Motor cortex stimulation for central and neuropathic facial pain: a prospective study of 10 patients and observations of enhanced sensory and motor function during stimulation. Neurosurgery 2005;56:290–7. discussion 290–7.
Canavero S, Bonicalzi V, Dotta M, Vighetti S, Asteggiano G, Cocito D. Transcranial magnetic cortical stimulation relieves central pain.Stereotact Funct Neurosurg 2002;78:192–6.
Carroll D, Joint C, Maartens N, Shlugman D, Stein J, Aziz TZ. Motor cortex stimulation for chronic neuropathic pain: a preliminary study of 10 cases. Pain 2000;84:431–7.
Drouot X, Nguyen JP, Peschanski M, Lefaucheur JP. The antalgic efficacy of chronic motor cortex stimulation is related to sensory changes in the painful zone. Brain 2002;125:1660–4.
Ebel H, Rust D, Tronnier V, Boker D, Kunze S. Chronic precentral stimulation in trigeminal neuropathic pain. Acta Neurochir (Wien) 1996;138:1300–6.
Flor H, Elbert T, Muhlnickel W, Pantev C, Wienbruch C, Taub E.Cortical reorganization and phantom phenomena in congenital and traumatic upper-extremity amputees. Exp Brain Res 1998;119:205–12.
Fregni F, Boggio PS, Mansur CG, Wagner T, Ferreira MJ, Lima MC,et al. Transcranial direct current stimulation of the unaffected hemisphere in stroke patients. Neuroreport 2005a;16:1551–5.
Fregni F, Boggio PS, Nitsche M, Bermpohl F, Antal A, Feredoes E,et al. Anodal transcranial direct current stimulation of prefrontal cortex enhances working memory. Exp Brain Res 2005b;166:23–30.
Fregni F, Boggio PS, Nitsche M, Marcolin MA, Rigonatti SP,Pascual-Leone A. Treatment of major depression with transcranial direct current stimulation. Bipolar Disord, in press.
Fregni F, Thome-Souza S, Nitsche M, Freedman SD, Valente KD,Pascual-Leone A. A controlled clinical trial of cathodal DC polarization in patients with refractory epilepsy. Epilepsia 2006;47:335–42.
Garcia-Larrea L, Peyron R, Mertens P, Gregoire MC, Lavenne F,Bonnefoi F, et al. Positron emission tomography during motor cortex stimulation for pain control. Stereotact Funct Neurosurg 1997;68:141–8.
Garcia-Larrea L, Peyron R, Mertens P, Gregoire MC, Lavenne F, Le Bars D, et al. Electrical stimulation of motor cortex for pain control: a combined PET-scan and electrophysiological study. Pain 1999;83:259–73.
Helsinki, 1964. http://www.wma.net/e/policy/b3.htm.
Herregodts P, Stadnik T, De Ridder F, D’Haens J. Cortical stimu-lation for central neuropathic pain: 3-D surface MRI for easy determination of the motor cortex. Acta Neurochir Suppl 1995;64:132–5.
Huang YZ, Edwards MJ, Rounis E, Bhatia KP, Rothwell JC. Theta burst stimulation of the human motor cortex. Neuron 2005;45:201–6.
Hummel F, Celnik P, Giraux P, Floel A, Wu WH, Gerloff C, et al.Effects of non-invasive cortical stimulation on skilled motor function in chronic stroke. Brain 2005;128:490–9.
Iyer MB, Mattu U, Grafman J, Lomarev M, Sato S, Wassermann EM.Safety and cognitive effect of frontal DC brain polarization in healthy individuals. Neurology 2005;64:872–5.
Karl A, Birbaumer N, Lutzenberger W, Cohen LG, Flor H.Reorganization of motor and somatosensory cortex in upper extremity amputees with phantom limb pain. J Neurosci 2001;21:3609–18.
Khedr EM, Kotb H, Kamel NF, Ahmed MA, Sadek R, Rothwell JC.Long lasting antalgic effects of daily sessions of repetitive trans-cranial magnetic stimulation in central and peripheral neuropathic pain. J Neurol Neurosurg Psychiatr 2005;76:833–8.
Lang N, Siebner HR, Ward NS, Lee L, Nitsche MA, Paulus W, et al.How does transcranial DC stimulation of the primary motor cortex alter regional neuronal activity in the human brain? Eur J Neurosci 2005;22:495–504.
Lefaucheur JP, Drouot X, Keravel Y, Nguyen JP. Pain relief induced by repetitive transcranial magnetic stimulation of precentral cortex.Neuroreport 2001;12:2963–5.
Lefaucheur JP, Drouot X, Menard-Lefaucheur I, Zerah F, Bendib B,Cesaro P, et al. Neurogenic pain relief by repetitive transcranial magnetic cortical stimulation depends on the origin and the site of pain. J Neurol Neurosurg Psychiatr 2004;75:612–6.
Liebetanz D, Nitsche MA, Tergau F, Paulus W. Pharmacological approach to the mechanisms of transcranial DC-stimulation-induced after-effects of human motor cortex excitability. Brain 2002;125:2238–47.
Migita K, Uozumi T, Arita K, Monden S. Transcranial magnetic coil stimulation of motor cortex in patients with central pain. Neuro-surgery 1995;36:1037–9. discussion 1039–40.
Nguyen JP, Lefaucheur JP, Decq P, Uchiyama T, Carpentier A,Fontaine D, et al. Chronic motor cortex stimulation in the treatment of central and neuropathic pain. Correlations between clinical, electrophysiological and anatomical data. Pain 1999;82:245–51.
Nicholson BD. Evaluation and treatment of central pain syndromes.Neurology 2004;62:S30–6.
Nitsche MA, Paulus W. Sustained excitability elevations induced by transcranial DC motor cortex stimulation in humans. Neurology 2001;57:1899–901.
Nitsche MA, Liebetanz D, Antal A, Lang N, Tergau F, Paulus W.Modulation of cortical excitability by weak direct current stimu-lation–technical, safety and functional aspects. Suppl Clin Neuro-physiol 2003a;56:255–76.
Nitsche MA, Schauenburg A, Lang N, Liebetanz D, Exner C, Paulus W, et al. Facilitation of implicit motor learning by weak transcra-nial direct current stimulation of the primary motor cortex in the human. J Cogn Neurosci 2003b;15:619–26.
Nitsche MA, Niehaus L, Hoffmann KT, Hengst S, Liebetanz D,Paulus W, et al. MRI study of human brain exposed to weak direct current stimulation of the frontal cortex. Clin Neurophysiol 2004;115:2419–23.
Nitsche MA, Seeber A, Frommann K, Klein CC, Rochford C, Nitsche MS, et al. Modulating parameters of excitability during and after transcranial direct current stimulation of the human motor cortex.J Physiol 2005;568:291–303.
Nuti C, Peyron R, Garcia-Larrea L, Brunon J, Laurent B, Sindou M,et al. Motor cortex stimulation for refractory neuropathic pain:four year outcome and predictors of efficacy. Pain 2005;118:43–52.
Peto R. Clinical trial methodology. Biomedicine 1978;28:24–36.
Peyron R, Garcia-Larrea L, Deiber MP, Cinotti L, Convers P, Sindou M, et al. Electrical stimulation of precentral cortical area in the treatment of central pain: electrophysiological and PET study. Pain 1995;62:275–86.
Pleger B, Janssen F, Schwenkreis P, Volker B, Maier C, Tegenthoff M.Repetitive transcranial magnetic stimulation of the motor cortex attenuates pain perception in complex regional pain syndrome type I. Neurosci Lett 2004;356:87–90. Pocock SJ. Allocation of patients to treatment in clinical trials.Biometrics 1979;35:183–97.
Romero JR, Anschel D, Sparing R, Gangitano M, Pascual-Leone A.Subthreshold low frequency repetitive transcranial magnetic stim-ulation selectively decreases facilitation in the motor cortex. Clin Neurophysiol 2002;113:101–7.
Ruck A, Sylven C. ‘‘Improvement’’ in the placebo group could be due to regression to the mean as well as to sociobiologic factors. Am J Cardiol 2006;97:152–3.
Strafella AP, Vanderwerf Y, Sadikot AF. Transcranial magnetic stimulation of the human motor cortex influences the neuronal activity of subthalamic nucleus. Eur J Neurosci 2004;20(8):2245–9.
Tsubokawa T, Katayama Y, Yamamoto T, Hirayama T, Koyama S.Chronic motor cortex stimulation in patients with thalamic pain.J Neurosurg 1993;78:393–401.
van Belle G, Uhlmann RF, Hughes JP, Larson EB. Reliability of estimates of changes in mental status test performance in senile dementia of the Alzheimer type. J Clin Epidemiol 1990;43:589–95.
Wallace BA, Ashkan K, Benabid AL. Deep brain stimulation for the treatment of chronic, intractable pain. Neurosurg Clin N Am 2004;15:343–57. vii.